Coming Full Circle
Thursday, November 1, 2018
Scientists find themselves reunited at OSU as they work on projects to help NASA get to Mars
By Shannon Rigsby
Eighteen years ago, a physicist and a materials scientist from different parts of the country were paired on a project for NASA involving the study of radiation. Their paths crossed again in 2008 as colleagues at Oklahoma State University.
Nearly two decades after their first NASA project, physicist Dr. Eric Benton, materials scientist Dr. Ranji Vaidyanathan and their graduate students are making the dream of reaching Mars safely a greater possibility for NASA.
Benton studies radiation itself, designing efficient, economical ways to accurately measure cosmic rays while Vaidyanathan creates new composite materials in hopes of protecting astronauts from them.
“Now, the new administration is talking about a ‘hop, skip and jump,’” Vaidyanathan said. “They are talking about hopping to the moon and jumping to Mars. Not a direct jump. The moon would come first, and creating a colony there. On the moon, they need to create what is called an igloo for protecting astronauts from space radiation and then jumping from there to Mars and back.”
Getting to Mars would take a year at today’s space travel speeds, Vaidyanathan said, noting the voyage isn’t even possible with current spacecraft materials. There’s no clear picture of exactly how much radiation astronauts are exposed to, nor is there a material for the space craft that would fully protect them.
That’s changing.
In partnership with NASA, Benton recently put an inexpensive radiation detector on the International Space Station to find out just how much radiation astronauts in space receive. Next spring, Vaidyanathan will have a composite material with a greater radiation shielding capability on the ISS as well.
One cell at a time
“Most of the dangerous radiation comes from outside the solar system,” Benton said. “It's called galactic cosmic rays that come from supernova events and huge stellar catastrophes like collisions of neutron stars.”
NASA scientists are most concerned about astronauts exposed to ionized radiation during long space missions. Ionized radiation damages cells, including causing genetic mutations that can lead to cancer.
“If you want to know what the radiation environment at 20,000 feet is, there’s some data but not much,” Benton said. “Is radiation a health problem to pilots? Probably not, but we don’t have a lot of data.”
The earth is protected by a shroud of atmosphere, an umbrella of moisture around 10 meters or about 33 feet thick, stopping the lion’s share of the radiation from space. The higher the altitude, the more radiation gets through. But how much? And what effect does it have on a human body?
“At the International Space Station, there’s nothing but the walls of the space station or things present inside like tanks of water to stop the radiation,” Benton said. “They get considerably more radiation. It’s not dangerous in the immediate sense, but it’s a slow, chronic accumulation. The most prominent health risk associated is an increased risk of cancer. Then there may be other effects as well. Chronic radiation exposure is considered to be one of the three biggest obstacles to human exploration and space flight.”
NASA’s current radiation detectors were developed long ago and cost millions of dollars. Benton and graduate students in his lab have been developing tissue-equivalent radiation dosimeters to be used in space for more than a decade. A $100,000 grant in 2017 from the NASA Experimental Program to Stimulate Competitive Research (NASA EPSCoR) program, matched with $50,000 in OSU funding, opened the door to send the latest version, the Active Tissue Equivalent Dosimeter, or ATED, into space.
Oklahoma’s NASA EPSCoR program is operated in conjunction with the Oklahoma Space Grant at OSU. The program allows Oklahoma researchers to compete for NASA funding with programs that advance scientific knowledge in areas important to the agency while diversifying the geographic reach of its investments.
“The fact that OSU teams are leading two of the 17 projects funded by the NASA EPSCoR program to be carried out on the International Space Station is a testament to the high-level science going on at OSU,” said Dr. Kenneth Sewell, OSU vice president for research. “OSU has a long history of partnership with NASA, and these projects extend that legacy into the future.”
The detector’s design includes a plastic ball inside a vacuum-packed canister that is refilled with a gas combination that mimics human tissue. The detector is the equivalent of one enlarged human cell. The ATED measures the simulated absorption of ionizing radiation by living tissue. The dosimeter can record data on different energy densities passing through the instrument, which has a similar response to radiation as living cells.
“What’s measured is the absorbed dose of energy deposited in the detector by radiation. We convert that measure to a biologically weighted dose equivalent for different types of radiation with different biological effects,” Benton said. “If this energy were instead passing through a cell, it would damage the cell.”
Adherence concerns
When he got the stamp of approval from NASA, Benton and graduate student Oliver Causey began modifying the unit to adhere to space station standards. Weight and power demands are premium concerns on spacecraft, so the instrument was designed with low-cost, lightweight components that need only tiny amounts of power. The ATED is doing its job better than competing technologies at a fraction of the cost, Benton said.
“Ours is sort of a better, stronger, faster, cheaper version of what NASA already has,” he said.
There were multiple requirements for the ISS version of ATED. If something malfunctioned, the unit had to shut down on its own without emitting any smoke or odor. The outer casing could have only rounded corners. Benton traveled to Johnson Space Center in Houston to put the ATED in a room full of antennae to ensure it wouldn’t broadcast radio waves that would interfere with the space station’s ability to communicate with ground control.
In November 2017, NASA gave its stamp of approval, and Benton shipped the ATED unit to Houston. It went into storage at Johnson Space Center, waiting to be packed in a space capsule for launch. Meanwhile, Benton had the engineering unit in Japan, calibrating it with heavy ions at one of only a handful of particle accelerators on earth that can produce the type of radiation astronauts will encounter in space.
“While I was in Japan, the detector stopped working,” Benton said. “The canister has to be pressurized near vacuum, and it leaked. I knew the one at the space center must have a design flaw.”
Benton called NASA and requested it be returned so the flaw could be fixed before launch. It went on a delivery truck on Friday, and Benton was supposed to have it the next business day. Monday came and went. The following Friday, Benton called NASA. They looked up the shipment only to discover the truck the ATED was on was destroyed in a fiery roadside crash en route from Tulsa to Stillwater.
Benton and Causey immediately went to work remaking their engineering unit, applying what they learned building the previous model to meet space station specifications.
We rebuilt it as quickly as we possibly could,” Benton said. “We gutted the engineering unit. Oliver Causey worked night and day.”
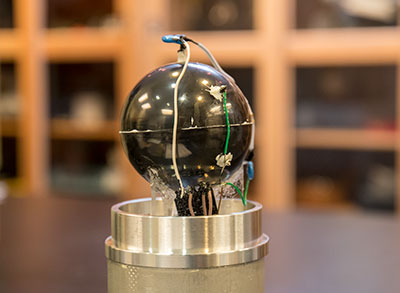
The rebuilt unit, now suitable for space, was put on another truck to NASA. On May 21, 2018, it was launched in the nose of a rocket and was installed in the ISS over the summer. Because of the budget and safety constraints, the unit is relatively primitive, unable to send data down on its own, Benton said. Radiation information is stored on a memory card like the one used in cameras and removed periodically by an astronaut who downloads the information to a laptop to transmit the information back to earth.
The day Causey defended his doctoral dissertation, he and Benton returned later to Oklahoma State University’s Venture 1 lab. In Benton’s email inbox was the first set of data ATED collected in space.
“(Causey) was thrilled,” Benton said. “He wanted to go home and celebrate. I told him, ‘No. Look at the data and see if it’s working. I have to know.’”
Using the data, the pair could follow the space station’s orbit, with spikes in radiation at the poles. As the space station passed over the South Atlantic Anomaly, where the radiation belt dips closest to the earth’s surface, the graph jumped predictably every time.
ATED worked, and it worked beautifully.
“It was good, clean, solid data,” Benton said. “That was 90 percent of the objective. We can start teasing out more subtle things but the first goal, did it give us the data we expected? Yes. It worked. ‘’
After Causey graduated, graduate student Bryan Hayes took up the cause and is working on a detailed analysis of the data from the ATED’s time in space. Hayes is also taking the lead on another ATED, an improved version, that should make it to the ISS in late 2019 or 2020.
It’s a puzzle Benton and his students are trying to complete — placing missing pieces in a sparse map of mankind’s knowledge of the levels and effects of radiation at different altitudes. And the problem isn’t relegated to space. There are questions to be answered in moving up through the atmosphere at all levels from the surface of the earth.
“This is a stepping stone to the whole program,” he said. “You’re trying to develop an inexpensive detector that’s easy to use and requires low power. My hope, ultimately, is to develop a device that we can put on UAVs, drones, balloons — to drop it in there and let it fly and collect as much data as we can and then start teasing out the picture.”
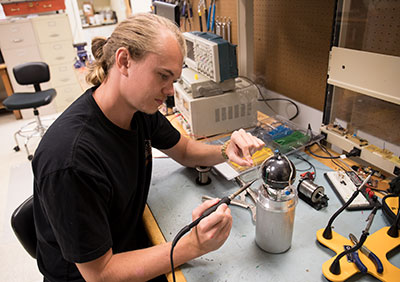
Radiation shield
While Benton works to measure and catalogue radiation, Ranji Vaidyanathan hopes to create a material that can replace aluminum as the primary building block of a spacecraft. While aluminum is considered lightweight, it doesn’t block radiation well.
Vaidyanathan thought of a composite that included hydrogen.
“I came up with an idea — I didn’t know if it was going to work,” he said. “The best material for radiation shielding other than water is polyethylene, a plastic. It has the highest hydrogen content in it. Hydrogen has no neutrons. Even if the hydrogen atom is hit by cosmic radiation, it is not going to release more energetic particles from that.”
Around 2013, Vaidyanathan decided to develop a composite material for radiation shielding that could double as the outer skin of a spacecraft. Polyethylene has a low melting point, much like a plastic bag. But when mixed with boron nitride, the melting temperature rises, and the resulting substance is harder than simple polyethylene. Vaidyanathan takes that composite and coats the outside with carbon fibers glued with expoxy.
Based on theoretical models developed by the Benton group, Vaidyanathan and Benton may have successfully come up with a solution — and at half the weight of aluminum.
“If you do not have proper protection, you cannot do a Mars mission with the current spacecraft materials,” Vaidyanathan said. “And every pound of material we have to send to space costs $8,000 to $10,000 per pound. Aluminum weighs 2.7 grams per centimeter cubed. But the composite is 1.5, almost half of that. If you have a spacecraft material made out of composite that can also protect astronauts at the same time from radiation — that is what NASA wants.”
Vaidyanathan worked for a company in the late 1990s on the cusp of 3D printing, where one nozzle sprays the working material and another sprays a support material that can later be washed off to reveal the multidimensional creation. Two decades later the filaments, or slender, threadlike fibers emitted by the printer, inspired him.
“I had the idea, if you have two materials you can do winding of two materials and you can line a composite tank and then wind it outside with the carbon fiber. We tried to do that, but we were never able to make the filament.”
By chance, Vaidyanathan discovered a student startup company in Wisconsin with the technology for creating filaments. It took six months for him to get the equipment, which arrived in May. Graduate student Lynsey Baxter came to work for Vaidyanathan for the summer in June. On the first day, he asked if she could create boron nitride polyethylene filaments with the machine.
“I expected her to take the whole two months to do this,” he said. “She came to me at the end of the day and said, ‘Now what do you want me to do?’ I said, ‘Make enough of the material to make a composite tank.’”
A spacecraft is, in its essence, a pressurized vessel, like a tank that holds natural gas. Vaidyanathan and his graduate students, Baxter and Korey Herrman, created a pressurized tank that Benton took to Japan.
“He does the material development, and I do all the testing and modeling from the radiation point of view,” Benton said. “We put it in the particle accelerator in Japan and with water in it, it stops all the radiation. We now have a material we are pretty happy with — SC2020 or space composite 2020.”
Benton and Vaidyanathan have secured some significant real estate aboard the ISS. A 3-by-3-inch piece of SC2020 that’s one inch thick will be secured to an experiment field that stays outside the space station called Materials on ISS Experiment, or MISSE. Pebbles used to measure absorbed radiation will be embedded at different depths in the piece, increasing the amount of data that can be collected. Along with the SC2020, OSU will have additional experimental pieces of 1.5-by-1.5-inch pieces of aluminum and copper, items with known degradation rates that will function as experiment controls. A 1.5-by-1.5-inch sample of polyethylene plastic, one inch thick will also be added as a comparison sample to the SC2020.
“This gives us the best picture we will ever get short of building a spacecraft out of it and flying it,” Benton said.
SC2020 will be delivered to NASA in February. It should launch in April, spend six months exposed to space radiation and then be returned to Benton and Vaidyanathan as early as November 2019.
Should the results they receive in a little less than a year be as promising as the testing so far, the implications could be as significant as putting a landing on Mars within closer reach.
“If you could line the whole spacecraft with these bottles of water made with SC2020, the astronauts will not be affected by radiation at all,” Vaidyanathan said. “We have one of the radiation shielding projects that NASA is considering. If we prove something today, it can go into a NASA spacecraft tomorrow. They don’t have to wait 20 years.”
For Benton and Vaidyanathan, life has come full circle. A relationship centered around scientific discovery that got its start at NASA nearly 20 years ago continues to lay foundations for more extensive and safer spaceflight.